Car Aerodynamics Basics and How-To Design Tips
Aerodynamics is the science of how air flows around and inside objects. More generally, it can be labeled “Fluid Dynamics” because air is really just a very thin type of fluid. Above slow speeds, the air flow around and through a vehicle begins to have a more pronounced effect on the acceleration, top speed, fuel efficiency and handling.
Therefore, to build the best possible car we need to understand and optimize how the air flows around and through the body, its openings and its aerodynamic devices.
Aerodynamic Principles
Drag
No matter how slowly a car is going, it takes some energy to move the car through the air. This energy is used to overcome a force called Drag.
Drag, in vehicle aerodynamics, is comprised primarily of three forces:
- Frontal pressure, or the effect created by a vehicle body pushing air out of the way.
- Rear vacuum, or the effect created by air not being able to fill the hole left by the vehicle body.
- Boundary layer, or the effect of friction created by slow moving air at the surface of the vehicle body.
Between these three forces, we can describe most of the interactions of the airflow with a vehicle body.
Frontal Pressure
Frontal pressure is caused by the air attempting to flow around the front of the vehicle as shown in diagram D1 below.
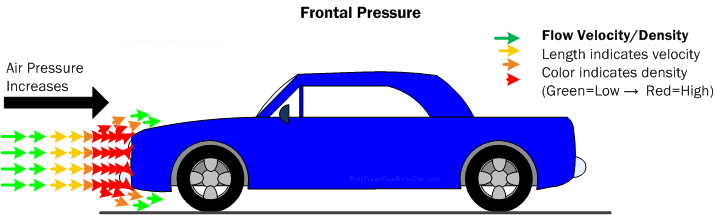
Diagram D1. Frontal Pressure is a form of drag where the vehicle must push air molecules out of the way as it travels through the air.
As millions of air molecules approach the front of the car, they begin to compress, and in doing so raise the air pressure in front of the car. At the same time, the air molecules travelling along the sides of the car are at atmospheric pressure, a lower pressure compared to the molecules at the front of the car.
Just like an air tank, if the valve to the lower pressure atmosphere outside the tank is opened, the air molecules will naturally flow to the lower pressure area, eventually equalizing the pressure inside and outside the tank. The same rules apply to any vehicle. The compressed molecules of air naturally seek a way out of the high pressure zone in front of the vehicle, and they find it around the sides, top and bottom of the vehicle as demonstrated in diagram D1.
Rear Vacuum
Rear vacuum is caused by the “hole” left in the air as a vehicle passes through it. To visualize this, let’s take a look at our demonstration car in diagram D2 below. As it drives down a road, the blocky sedan shape of the car creates a hole in the air. The air rushes around the body as described above.
At speeds above a crawl, the space immediately behind the car’s rear window and trunk is “empty” or like a vacuum. These empty areas are the result of the air molecules not being able to fill the hole as quickly as the car can make it. The air molecules attempt to fill in to this area, but the car is always one step ahead, and as a result, a continuous vacuum sucks in the opposite direction of the car.
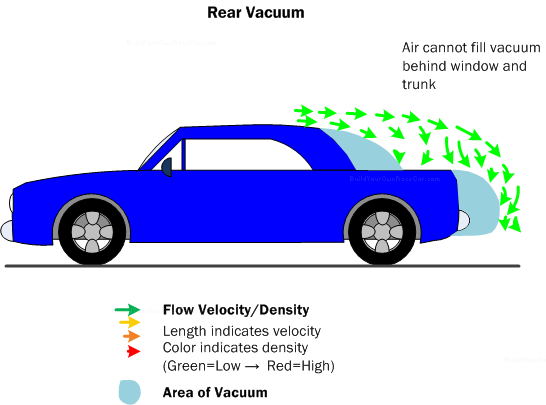
Diagram D2. Rear Vacuum (Also known as flow detachment) is another form of drag where the air the vehicle is passing through cannot fill the space of the hole left behind by the vehicle, leading to what amounts to a vacuum.
This inability to fill the hole left by the car is technically called Flow detachment.
Flow detachment applies only to the “rear vacuum” portion of the drag forces and has a greater and greater negative effect as vehicle speed increases. In fact, the drag increase with the square of the vehicle speed, so more and more horsepower is needed to push a vehicle through the air as its speed rises.
Therefore, when a vehicle reaches high speeds it becomes important to design the car to limit areas of flow detachment. Ideally, we give the air molecules time to follow the contours of a car’s bodywork, and to fill the hole left by the vehicle, its tires, its suspension and its protrusions (i.e. mirrors, roll bars).
If you have witnessed the Le Mans race cars, you will have seen how the tails of these cars tend to extend well back of the rear wheels, and narrow when viewed from the side or top. This extra bodywork allows the air molecules to converge back into the vacuum smoothly along the body into the hole left by the car’s cockpit, and front area, instead of having to suddenly fill a large empty space.
The force created by the rear vacuum exceeds that created by frontal pressure, so there is very good reason to minimize the scale of the vacuum created at the rear of the vehicle.
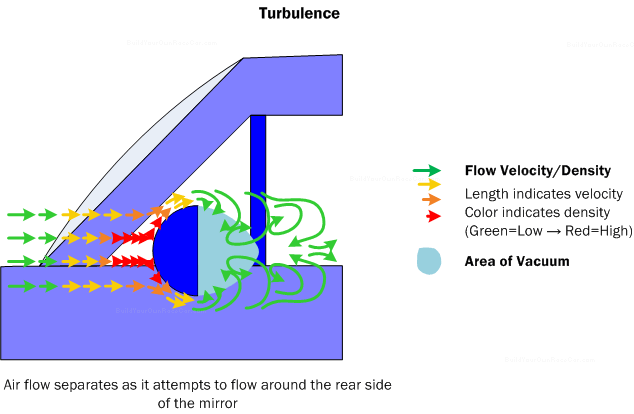
Diagram D3. Turbulence is created by the detachment of an air flow from the vehicle. The final unavoidable detachment at the very rear of the vehicle leaves a turbulent wake.
When the flow detaches, the air flow becomes very turbulent and chaotic when compared to the smooth flow on the front of an object.
If we look at a protrusion from the car such as the mirror in diagram D3 above, we see flow detachment and turbulence in action. The air flow detaches from the flat side of the mirror, which of course faces toward the back of the car.
The turbulence created by this detachment can then affect the air flow to parts of the car which lie behind the mirror. Intake ducts, for instance, function best when the air entering them flows smoothly. Wings generate far more downforce with smooth flows over them as well. Therefore, the entire length of the car really needs to be optimized (within reason) to provide the least amount of turbulence at high speed.
Drag Coefficient
To enable the comparison of the drag produced by one vehicle versus another, a dimensionless value called the Coefficient of Drag or Cd was created. Every vehicle has a Cd which can be measured using wind tunnel data. The Cd can be used in drag equations to determine the drag force at various speeds. In his comprehensive book “Race Car Aerodynamics: Designing for Speed“, Joseph Katz provides a table of common vehicles and their Cds and Frontal Areas. Here is an excerpt from that table:
Vehicle Type | Drag | Frontal area | |
Coefficient Cq | A[m2] | CDA [m2] | |
Ford Escort 1.3 GL | 0.39-0.41 | 1.83 | 0.71-0.75 |
Nissan Cherry GL | 0.39-0.41 | 1.83 | 0.71-0.75 |
Volvo 360 GLT | 0.40-0.41 | 1.95 | 0.78-0.80 |
Honda Accord 1.8 EX | 0.40-0.42 | 1.88 | 0.75-0.79 |
Nissan Stanza SGL 1.8 | 0.40-0.42 | 1.88 | 0.75-0.79 |
Mazda 323 1.5 | 0.41-0.43 | 1.78 | 0.73-0.77 |
Nissan Sunny | 0.41-0.43 | 1.82 | 0.75-0.78 |
Talbot Horizon GL | 0.41-0.44 | 1.85 | 0.76-0.81 |
Alfa Romeo Giulietta 1.6 | 0.42-0.44 | 1.87 | 0.79-0.82 |
Toyota Corolla 1300 DX | 0.45-0.46 | 1.76 | 0.79-0.81 |
VW Golf Cabrio GL | 0.48-0.49 | 1.86 | 0.89-0.91 |
Full-size sedans | |||
Renault 25 TS | 0.30-0.31 | 2.04 | 0.61-0.63 |
Audi 100 1.8 | 0.30-0.31 | 2.05 | 0.62-0.64 |
Mercedes 190 E (190 D) | 0.33-0.35 | 1.90 | 0.63-0.67 |
Mercedes 380 SEC | 0.34-0.35 | 2.10 | 0.71-0.74 |
Mercedes 280 SE | 0.36-0.37 | 2.15 | 0.77-0.80 |
Mercedes 500 SEL | 0.36-0.37 | 2.16 | 0.78-0.80 |
BMW 518i (520i, 525e) | 0.36-0.38 | 2.02 | 0.73-0.77 |
Citroen CX 25 Gti | 0.36-0.39 | 1.99 | 0.72-0.78 |
BMW 323i | 0.38-0.39 | 1.86 | 0.71-0.73 |
Alfa Romeo 90 2.0 | 0.38-0.40 | 1.95 | 0.74-0.78 |
Mazda 929 2.0 GLX | 0.39-0.44 | 1.93 | 0.75-0.85 |
Saab 900 Gli | 0.40-0.42 | 1.95 | 0.78-0.82 |
Volvo 740 GLE | 0.40-0.42 | 2.16 | 0.86-0.91 |
Volvo 760 Turbo w/intercooler | 0.40-0.42 | 2.16 | 0.86-0.91 |
Peugeot 505 STI | 0.41-0.43 | 1.97 | 0.81-0.85 |
Peugeot 604 STI | 0.41-0.43 | 2.05 | 0.84-0.88 |
BMW 728i (732i/735i) | 0.42-0.44 | 2.13 | 0.89-0.94 |
BMW 745i | 0.43-0.45 | 2.14 | 0.92-0.96 |
Ford Granada 2.3 GL | 0.44-0.46 | 2.13 | 0.94-0.98 |
Sports cars | |||
Porsche 924 | 0.31-0.33 | 1.80 | 0.56-0.59 |
Porsche 944 Turbo | 0.33-0.34 | 1.90 | 0.63-0.65 |
Nissan 300 ZX | 0.33-0.36 | 1.82 | 0.60-0.66 |
Mazda 626 Coupe | 0.34-0.36 | 1.88 | 0.64-0.68 |
Opel Monza GSE | 0.35-0.36 | 1.95 | 0.68-0.70 |
Renault Fuego GTX | 0.34-0.37 | 1.82 | 0.62-0.67 |
Honda CRX Coupe | 0.35-0.37 | 1.72 | 0.60-0.64 |
Audi Coupe GT 5E | 0.36-0.37 | 1.83 | 0.66-0.68 |
Chevrolet Corvette | 0.36-0.38 | 1.80 | 0.65-0.68 |
Chevrolet Camaro Z 28 E | 0.37-0.38 | 1.94 | 0.72-0.74 |
Mazda RX-7 | 0.36-0.39 | 1.69 | 0.61-0.66 |
Toyota Celica Supra 2.8i | 0.37-0.39 | 1.83 | 0.68-0.71 |
VW Scirocco GTX | 0.38-0.39 | 1.74 | 0.66-0.68 |
Porsche 911 Carrera | 0.38-0.39 | 1.78 | 0.68-0.69 |
Honda Prelude | 0.38-0.40 | 1.84 | 0.70-0.74 |
Mitsubishi Starion Turbo | 0.38-0.40 | 1.84 | 0.70-0.74 |
Porsche 928 S | 0.38-0.40 | 1.96 | 0.74-0.78 |
Porsche 911 Carrera Cabrio | 0.40-0.41 | 1.77 | 0.71-0.73 |
Jaguar XJ-S | 0.40-0.41 | 1.92 | 0.77-0.79 |
From this table and our knowledge of the body shape of some of these vehicles, we can conclude that the best Cd is achieved when a vehicle has these attributes:
- Has a small nose/grill, to minimize frontal pressure.
- Has minimal ground clearance below the grill, to minimize air flow under the car.
- Has a steeply raked windshield (if any) to avoid pressure build up in front.
- Has a “Fastback” style rear window/deck or sloped bodywork, to permit the air flow to stay attached.
- Has a converging “Tail” to keep the air flow attached, and to minimize the area against which flow detachment eventually occurs
If it sounds like we’ve just described a sports car, you’re right. In truth though, to be ideal, a car body would be shaped like a tear drop, as even the best sports cars experience flow detachment. However, tear drop shapes are not conducive to the area where a car operates, and that is close to the ground. Airplanes don’t have this limitation, and therefore teardrop shapes work.
The best road cars today manage a Cd of about 0.28. Formula 1 cars, with their wings and open wheels (a massive drag component) manage a minimum of about 0.75.
If we consider that a flat plate has a Cd of about 1.0, an F1 car really seems inefficient, but what an F1 car lacks in aerodynamic drag efficiency, it makes up for in downforce and horsepower.
Aerodynamics How-To Tips (1/4)
Cover Open wheels
Open wheels create a great deal of drag and air flow turbulence, similar to the diagram of the mirror in the “Turbulence” section above. Full covering bodywork is probably the best solution, if legal by regulations, but if partial bodywork is permitted, placing a converging fairing behind the wheel provides maximum benefit.
Minimize Frontal Area
The smaller the hole your car punches through the air, the better it will accelerate, the higher the top speed, and the lower the fuel consumption it will have. It is usually much easier to reduce FA (frontal area) than the Cd (Drag coefficient).
Converge Bodywork Slowly
Bodywork which quickly converges or is simply truncated, forces the air flow into turbulence, and generates a great deal of drag. As mentioned above, it also can affect aerodynamic devices and bodywork further behind on the vehicle body.
49 2 36 7